ASSIGNMENT SHEET
BASIC THERMODYNAMICS
Assignment Sheet Number 60B-101
INTRODUCTION
A basic engineering background is needed to understand the operation of a ship. This is not limited to the operation of propulsion, but encompasses systems throughout the ship that the entire crew uses on a day to day basis. Knowledge of thermodynamic and fluid systems form the foundation of understanding the operation of the various systems found on all surface ships.
LESSON TOPIC LEARNING OBJECTIVES
Terminal Objective
2.0 Explain the fundamentals and principles of thermodynamics and fluid mechanics in relation to the design, construction, and operation of engineering plant equipment.
Enabling Objectives
2.1 Explain the First and Second Laws of Thermodynamics.
2.2 Define the following with respect to thermodynamics:
- Potential energy
- Kinetic energy
- Internal energy
- Chemical energy
2.3 Define the following:
- Heat
- Mechanical work
- Flow work
2.4 Define the following methods of heat transfer and the mechanics of each:
- Conduction
- Convection
- Radiation
2.5 Define the following as they apply to a thermodynamic system:
- Sensible heat
- Saturation point
- Saturated liquid
- Superheated steam (dry steam)
- Saturated steam (wet steam)
- Desuperheated steam
- Subcooled liquid
- Latent heat
- State
- Property
2.6 Define the thermodynamic cycle and state the five basic elements necessary to support the cycle as it applies to the following types of propulsion plant:
- Steam
- Gas Turbine
- Diesel
2.7 UNASSIGNED, reserved for future use.
2.8 UNASSIGNED, reserved for future use.
2.9 UNASSIGNED, reserved for future use.
STUDY ASSIGNMENT
- Read Information Sheet 60B-101.
- Outline Information Sheet 60B-101 using the enabling objectives for lesson 62B-101 as a guide.
- Answer study scenarios.
STUDY SCENARIOS
You are assigned as Auxiliaries Officer on board USS LEYTE GULF (CG 55), currently undergoing a repair availability in Mayport FL. One evening, while studying for your upcoming SWO board with the other junior officers in the duty section, some questions come up on engineering theory.
- What does the Second Law of Thermodynamics imply about the efficiency of naval propulsion plants?
- What type of steam is produced if saturated steam is heated past the saturation temperature at a constant pressure? What type of heat are you adding, latent or sensible?
The next morning, you observe shipyard workers conducting an open and inspect of a shell-and-tube heat exchanger.
- What types of heat transfer occur in this type of heat exchanger?
The inspection reveals a large amount of scale on the tubes of the heat exchanger. The shipyard workers tell you that this will not affect the efficiency of the heat exchanger, and that the tubes do not require cleaning.
- Should you agree with the shipyard workers? Why or why not?
INFORMATION SHEET
BASIC THERMODYNAMICS
Information Sheet Number 60B-101
INTRODUCTION
A basic engineering background is needed to understand the operation of a ship. This is not limited to the operation of propulsion, but encompasses systems throughout the ship that the entire crew uses on a day to day basis. Knowledge of thermodynamic and fluid systems form the foundation of understanding the operation of the various systems found on all surface ships.
REFERENCES
- Introduction to Naval Engineering (Naval Institute Press, 1985), Chapters 2 & 3
- Principles of Naval Engineering (NAVPERS 10788-B), Chapters 7 & 8
- Elements of Applied Thermodynamics (Naval Institute Press) Chapters 1 & 7
INFORMATION
- Definitions
- Energy - The capacity for producing an effect or doing work.
- Forms of Energy
- Stored
- Potential energy - energy stored by virtue of stresses existing in a system. Commonly related to the work done against gravity in elevating the object from some arbitrary reference plane to some higher level.
- Internal energy - the energy in a substance associated with the random motion, rotation, and vibrations of a substance’s molecules. Thermal energy is the stored internal energy within a substance.
- Kinetic energy - the energy in a substance that is dependent upon the body’s mass and velocity.
- Chemical energy - the energy stored in chemical bonds that is released in chemical reactions.
- Flow work - the energy necessary to maintain a steady flow of a stream of fluid.
- Energy in Transition
- Heat - energy which is transferred from one region to another by reason of a temperature difference.
- Mechanical work - force applied through a distance.
- Fundamental Laws of Thermodynamics
- The First Law of Thermodynamics (Conservation of Energy) - Energy can be neither created nor destroyed, but only transformed. The heat from the burning fuel in our engines and boilers does nothing to turn the propeller shafts. It must be applied to turbines or pistons to make them move, and they in turn will spin the shafts. We don’t gain any energy from this transformation. In financial terms, we can’t make a profit. In fact, we can’t break even, which leads to...
- The Second Law of Thermodynamics (Thermal Efficiency) - No engine, actual or ideal, can convert all the heat supplied to it into work. Some heat is lost (not converted to work) as it is applied to the turbines and pistons, and more is lost as they spin the propeller shafts. It has to go somewhere, though, and it does. This lost heat becomes friction of the moving metal parts, and it must be carried away by cooling systems.
- There are three important rules to remember about heat and work:
- Heat and work are the same thing. Both are forms of energy, but heat is usually the input to the engine or boiler (from burning fuel) and work is the output (rotary motion).
- You can always change work to heat but you cannot change all the heat to work. Some of the heat that was supposed to become work is always lost as friction, and friction is another energy output from the engine or boiler.
- Heat only flows from a hot place to a cold place. This fact is why we have an engineering plant. We need to take heat from where we don’t want it (e.g. on our moving metal parts) and put it where we do want it (off the ship), and at the same time transform as much of it as possible to work (spin the shafts). Even in the refrigeration plant, where heat moves from a cold place (inside the chill box) to a hot place (outside the chill box), we need to put more energy into the system (with a compressor) to move it.
- Thermodynamic Cycle
- Put all the above information together. We need to move heat around in a certain direction and we need to get work from it. We do this through the thermodynamic cycle, which requires five elements:
- First we need a working substance, to carry the energy.
- Next we need a pump, to move the working substance.
- Third, we need a high temperature region, provided by burning fuel. This adds energy to the working substance.
- Fourth, we need an engine to convert that added energy into work, and we know that some of that energy will be lost as friction.
- The fifth element is a low temperature region to draw the heat of friction away from our moving metal parts, provided by our cooling systems.
- Heat Transfer
- In a boiler, combustion gases heat up the steam generating tubes, and this heat boils water to the steam that will drive the plant. In diesel and gas turbine engines, intake air is heated by combustion and then transfers this energy to moving parts, eventually producing work in the form of rotary motion.
- Experience has taught us that unintended heat transfer also takes place in an engineering plant. Many pipes in the main spaces are lagged (insulated), because the hot fluids (e.g. steam, hot water) they are carrying would transfer a lot of heat to the spaces themselves. When the ship sits pierside at Newport in December, with cold water in direct contact with the hull and most of the plant secured, the main space could get chilly.
- There are three methods of heat transfer.
- Conduction is the flow of heat between two regions in physical contact. Stirring spaghetti in boiling water with a steel ladle is a good (and painful) introduction to the world of conduction.
- Radiation is the flow of heat between two regions that are not in physical contact. If you are close enough to the pot to stir the spaghetti, you probably feel the warmth from the stove. The air between you and the stove is not getting warmer, but you are.
- Any pipe carrying steam will get hot. The steam and metal are in physical contact and the heat passes to the metal by conduction. If the pipe is unlagged, the metal will radiate heat into the space, where objects (including thermometers) and people will get warmer even though the air will not.
- Convection is the transportation of one mass of fluid within another mass of fluid. Heat is carried along in convection, but not actually transferred unless by conduction or radiation. The air over a heater is itself warmed, and rises. The cooler surrounding air is drawn in to replace it, and now we have a convection current. In a cooling system, the cooling fluid (lube oil) is driven through piping by a pump and flows past the moving metal parts mentioned earlier. The heat on those parts is transferred to the fluid by conduction and carried away by convection.
- Use of Heat Exchangers
- Rarely is heat where we want it. In refrigeration systems it is inside the chill box and freeze box, and we want it out in the ocean. In lube oil systems it is either in the reduction gears and bearings, and we want it out in the ocean. Regardless of what our cooling liquid is, we use it to transfer heat from a hot place to somewhere that is even colder than the cooling liquid. This colder region is provided by the heat exchanger.
- Heat exchangers are where two fluids meet to transfer heat. The hotter fluid (which is carrying the heat from the moving metal parts) gives it up and cools down (and flows back to the moving metal parts to pick up more heat), the colder fluid receives it and warms up, and then carries it away. The most common type of heat exchanger is the tube-in-shell. One fluid is confined to the inside of a set of tubes, and the other fluid flows around the outside of these tubes and is confined within a shell that surrounds it all.
- There are three types of flow patterns with the tube-in-shell:
- In the Parallel Flow pattern, both fluids travel in the same direction for the length of the heat exchanger. Initially the temperature difference between the two fluids is high and heat transfer is efficient, but this drops off rapidly and the heat transfer becomes inefficient. One end of the heat exchanger is stressed by the very hot and very cold liquids passing through it, while the other isn’t; this uneven stress damages the exchanger over time. Parallel flow heat exchangers are uncommon in the fleet.
- In Counter Flow heat exchangers, the two fluids travel in opposite directions for the length of the heat exchanger. This keeps the temperature difference between the two fluids roughly the same even as the heat is transferred, so heat transfer efficiency doesn’t drop. Because the temperature difference is constant, the heat exchanger itself experiences an even stress along its entire length. Counter flow heat exchangers are very common in naval applications.
- In Cross Flow heat exchangers, the two fluids travel at angles to one another, and the volumetric rate of one is much greater than the other. These heat exchangers are often used to change the phase of one of the fluids, and in these cases, the heat transferred is latent heat. This means that the temperature of the phase-changing fluid does not change.
- Heat exchangers rely on the physical contact of two different temperature regions (i.e. conduction) to work. Film or scale build up on the tube walls reduces this physical contact dramatically and the efficiency of heat transfer drops. The hot fluid doesn’t get cooled enough, and when next it passes through the moving metal parts (which it is supposed to cool), it doesn’t pick up enough heat. Over time, the moving metal parts overheat and could break down. To detect this problem before it can damage our plant, we monitor the temperatures at the inlet and outlet of heat exchangers.
- Properties of Water and Steam
- In order to demonstrate how these principles of energy and heat transfer apply to a real-world system, we shall examine the process of changing water to steam.
- Definitions:
- Sensible heat - the heat added to a substance which produces a temperature increase.
- Latent heat - the heat added to a substance which causes a phase change.
- Saturation point - temperature at which a liquid turns to vapor or a vapor to liquid. This is dependent on the pressure on the liquid.
- Saturated steam - steam at saturation temperature for a given pressure (i.e., 490 F for 600 PSI); any more heat added will be used to convert more water to steam. Also commonly referred to as "wet steam."
- Superheated steam - steam above the saturation temperature for a given pressure. Any heat added will increase the temperature and internal energy of the steam. For example, steam is superheated in a 600 PSI boiler to 850 F. Commonly referred to as "dry stream."
- Desuperheated steam - superheated steam that has been cooled in a desuperheater. For 600 PSI systems, desuperheated steam’s temperature is around 650 F. It is still superheated, but to a lesser degree.
- When a teapot of water is placed on a stove’s hot burner, sensible heat begins to transfer into the water. This extra energy being added to the water raises the internal energy of the water. We see this rise in internal energy as a temperature increase. When the water reaches 212 F, the temperature stops rising. Continued heat addition is now in the form of latent heat. The energy we add with latent heat still increases the internal energy of the water, only now we see this increase as a change in the phase of the water (i.e., water to steam) rather than as a temperature increase.
- Types of Thermodynamic Cycles
- All types of naval propulsion plants are heat engines which convert heat energy to mechanical energy. There are five basic components of any heat engine which convert heat energy to mechanical energy or use mechanical energy to remove heat:
- Working substance. This is the medium by which energy is carried through a cycle.
- Engine. This is the device which converts the energy of a working substance into useful mechanical energy required to perform work.
- Heat source. The heat source supplies heat to the working substance.
- Heat sink. Heat exchangers function as heat sinks absorbing heat from the working substance.
- Pump. The system pump moves the working substance from the low pressure side to the high pressure side of the cycle
- Three major systems will be described in terms of their major components and overall system operation: steam (Rankine cycle), gas turbine (Brayton cycle), and diesel (Diesel cycle)
- Steam (Rankine cycle). The steam cycle uses water as a working substance. It is considered a closed cycle because the working substance is reused in the system. The Rankine Cycle is illustrated in Figure 1. Water is changed to steam in a boiler (heat source), then sent to turbines where mechanical work is extracted (engine). The steam then enters a condenser, where it is changed back into water (heat sink), then processed by the feed system and returned to the boiler via a feed pump (pump).
- Gas Turbine (Brayton cycle). Gas turbine engines use air as a working substance. Air is drawn into the gas turbine by a compressor (pump), where it is mixed with fuel and burned in a combustor (heat source), producing hot rapidly expanding combustion gases which turn a turbine (engine). The Brayton cycle is considered an open cycle, because the working substance is not reused, but given up to in the heat receiver (in this case, the exhaust gases are released into the atmosphere. Figure 2 is a cut away view of a basic gas turbine engine and some of its major components.
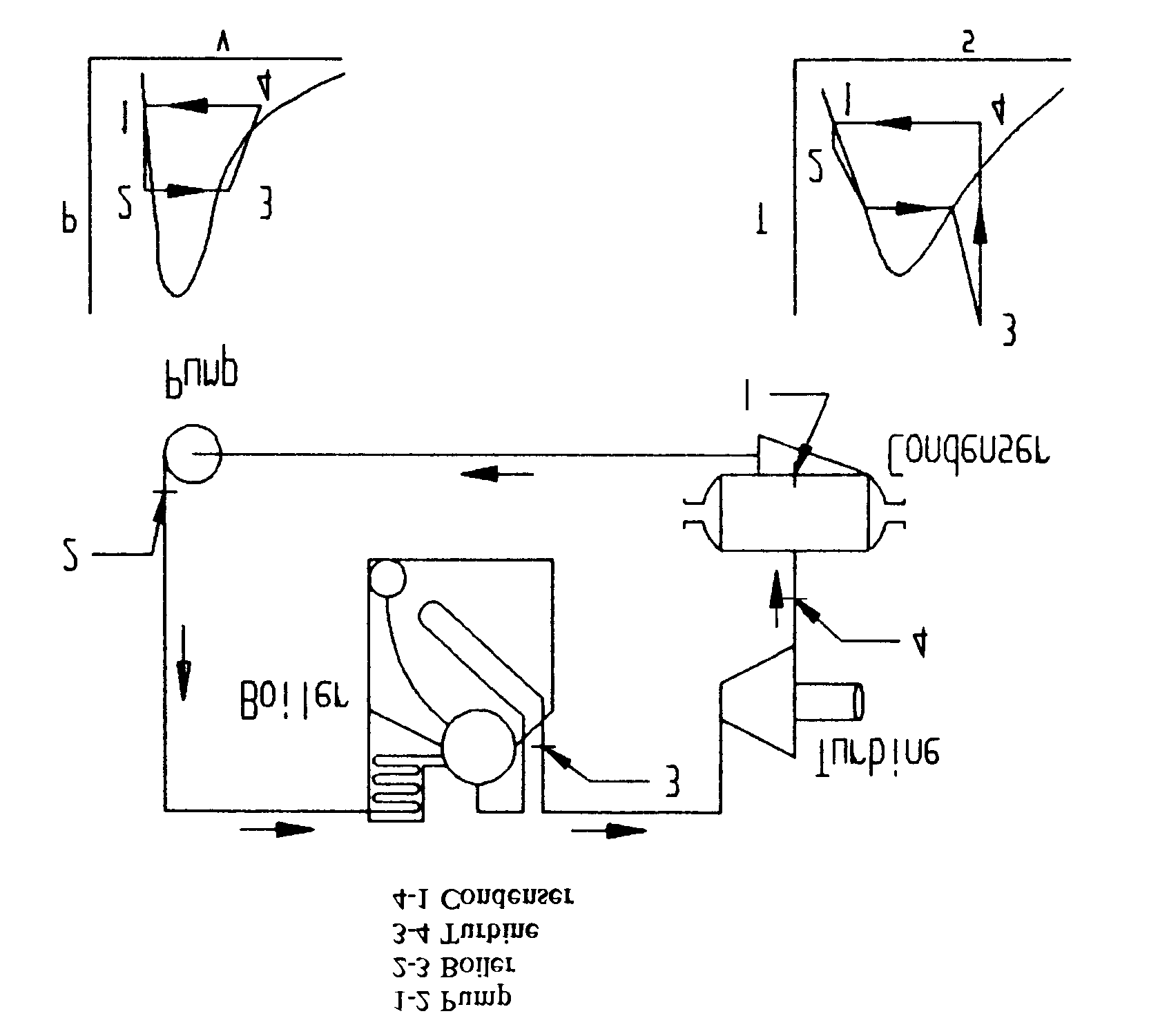
Figure 1 - Rankine Cycle (Steam)
Figure 2 - Gas Turbine
- Diesel Engines (Diesel cycle). Like gas turbine engines, diesel engines use an open cycle with air as the working substance. A diesel engine operates on the principle that the fuel will auto-ignite once injected into a cylinder of hot compressed air. A piston (pump) compresses air in a cylinder to a very high pressure which causes temperature of the air to rise to a high value due to the heat of compression. Fuel is then injected into the cylinder. The high temperature air is above the auto ignition temperature of the fuel and this will cause the air/fuel mixture to ignite (heat source). The resulting explosion will yield a piston power stroke which forces the crankshaft to turn (the crankshaft is attached directly to the output of the engine). Exhaust gases are released into the atmosphere (heat sink). Figure 3 is pressure-volume diagram illustrating the Diesel cycle.
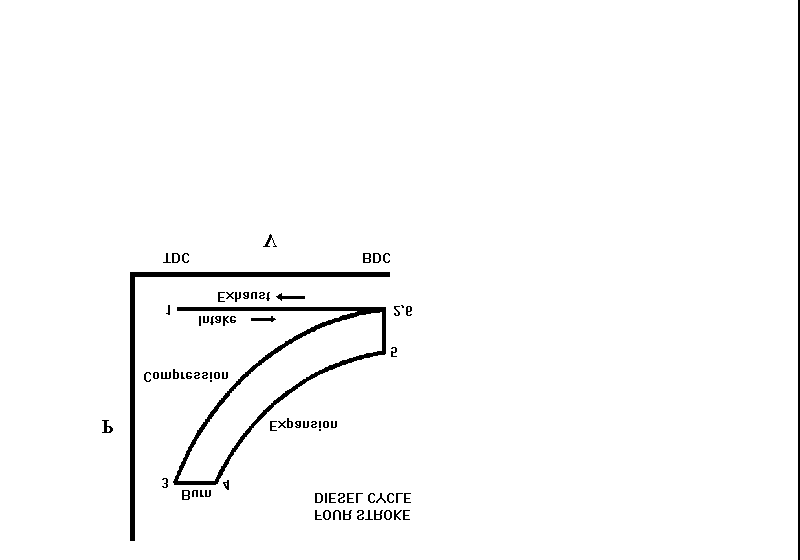
Figure 3 - Diesel Engine